Nanopore
|
Read other articles:

American writer and druid This article has multiple issues. Please help improve it or discuss these issues on the talk page. (Learn how and when to remove these template messages) This article may rely excessively on sources too closely associated with the subject, potentially preventing the article from being verifiable and neutral. Please help improve it by replacing them with more appropriate citations to reliable, independent, third-party sources. (February 2024) (Learn how and when to remov…

A Marked ManIklan surat kabarSutradaraJohn FordDitulis oleh John Ford (cerita) George Hively (skenario) PemeranHarry CareySinematograferJohn W. BrownDistributorUniversal Film Manufacturing CompanyTanggal rilis 19 Oktober 1917 (1917-10-19) Durasi50 menitNegaraAmerika SerikatBahasaAntarjudul Inggris A Marked Man adalah sebuah film koboi bisu Amerika tahun 1917 garapan John Ford dan menampilkan Harry Carey. Film tersebut dianggap menjadi film hilang.[1] Referensi ^ Progressive Silent F…

Навчально-науковий інститут інноваційних освітніх технологій Західноукраїнського національного університету Герб навчально-наукового інституту інноваційних освітніх технологій ЗУНУ Скорочена назва ННІІОТ ЗУНУ Основні дані Засновано 2013 Заклад Західноукраїнський на…
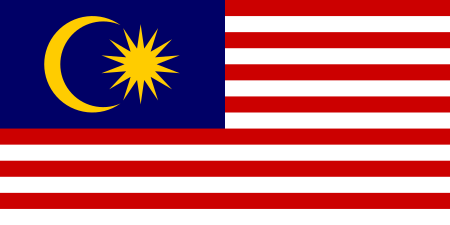
تشين بينغ معلومات شخصية الميلاد 22 أكتوبر 1924 فيرق الوفاة 16 سبتمبر 2013 (88 سنة) بانكوك مواطنة ماليزيا عضو في جيش الشعب الماليزي المناهض لليابان [لغات أخرى]، وجيش التحرير الوطني المالايوي الحياة العملية المهنة سياسي، وبارتيزان الحزب الحزب …

العلاقات الأردنية النمساوية الأردن النمسا الأردن النمسا تعديل مصدري - تعديل العلاقات الأردنية النمساوية هي العلاقات الثنائية التي تجمع بين الأردن والنمسا.[1][2][3][4][5] مقارنة بين البلدين هذه مقارنة عامة ومرجعية للدولتين: وجه المقارنة الأ…

Liquid (or partially liquid) food for animals For other uses, see Swill (disambiguation). Poster for pig swill for auction sale, London, 1877 Swill is liquid (or partially liquid) food for animals.[1] The term can also be used as a derogatory label for any drink meant for human ingestion, perceived as unpalatable or nearly so. Pig swill Pig swill, hog swill, or hogwash is kitchen refuse used to feed pigs.[2] Historically, pig farmers arranged collection of swill, e.g. by means of…

Varieties of the Aramaic language Neo-AramaicModern AramaicGeographicdistributionIraq, Iran, Syria, Turkey, Lebanon and the Assyrian diasporaLinguistic classificationAfro-AsiaticSemiticWest SemiticCentral SemiticNorthwest SemiticAramaicNeo-AramaicSubdivisions Western Neo-Aramaic Central Neo-Aramaic Northeastern Neo-Aramaic Neo-Mandaic Lebanese Aramaic† Glottologaram1259 (Aramaic) The Neo-Aramaic or Modern Aramaic languages are varieties of Aramaic that evolved during the late medieval an…

American cultural anthropologist, author (1924–1974) For other uses, see Ernest Becker (disambiguation). Ernest BeckerBorn(1924-09-27)September 27, 1924Springfield, Massachusetts, U.S.DiedMarch 6, 1974(1974-03-06) (aged 49)Burnaby, British Columbia, CanadaAlma materSyracuse University (BS, PhD)Known forDeath-centric perspective on human psychology, eliciting the creation of Terror Management TheoryNotable work The Birth and Death of Meaning (1971) The Denial of Death (1973) Esca…

Centre-left Norwegian political party Labour Party ArbeiderpartietAbbreviationA/ApLeaderJonas Gahr StøreParliamentary leaderRigmor AasrudFounded22 August 1887; 136 years ago (1887-08-22)HeadquartersYoungstorget 2 A, 5th floor, OsloYouth wingWorkers' Youth LeagueMembership (2023) 49,042[1]IdeologySocial democracyPolitical positionCentre-leftEuropean affiliationParty of European SocialistsInternational affiliationProgressive AllianceNordic affiliationSAMAKThe Social…

2018 ceremony of the Grammy Awards 60th Annual Grammy AwardsOfficial posterDateJanuary 28, 2018 (2018-01-28)7:30–11:00 p.m. ESTLocationMadison Square GardenNew York City, New YorkHosted byJames CordenMost awardsBruno Mars (6)Most nominationsJay-Z (8)Websitehttps://www.grammy.com/awards/60th-annual-grammy-awards-2017 Television/radio coverageNetworkCBSViewership19.8 million[1] ← 59th · Grammy Awards · 61st → The 60th Annual Gram…

Hanford MacNider Hanford MacNider (2 Oktober 1889 – 18 Februari 1968) adalah seorang perwira senior Angkatan Darat Amerika Serikat yang berjuang dalam dua perang dunia. MacNider menjabat sebagai diplomat, Asisten Menteri Perang Amerika Serikat, dari 1925 sampai 1928, dan Komandan Nasional Legiun Amerika dari 1921 sampai 1922. Pranala luar Cari tahu mengenai Hanford MacNider pada proyek-proyek Wikimedia lainnya: Gambar dan media dari Commons Entri basisdata #Q5647800 di Wikidata H…

لمعانٍ أخرى، طالع فرنسيسكو رودريغيز (توضيح). فرنسيسكو رودريغيز معلومات شخصية الميلاد 17 يونيو 1978 (46 سنة) المرية الطول 1.74 م (5 قدم 8 1⁄2 بوصة) مركز اللعب مهاجم الجنسية إسبانيا معلومات النادي النادي الحالي إلتشي (مدرب) مسيرة الشباب سنوات فريق 1995–1996…

Film production company, 1927 to 1970 British National Pictures redirects here. For a similarly named company, see British National Films Company. Associated British Picture CorporationIndustryFilm, televisionFounded1927Defunct1970FateFolded into EMI-ElstreeSuccessorEMI-ElstreeHeadquartersEnglandSubsidiaries Associated British Cinemas Ltd. Associated British Pathé Ltd. Associated British Cinemas (Television) Ltd. Associated British Productions Ltd. Associated British Picture Corporation (ABPC),…

البراء بن عازب تخطيط اسم الصَّحابي البراء بن عازب ملحوقًا بالترضي عنه - -. معلومات شخصية اسم الولادة البراء بن عازب الميلاد سنة 600 المدينة المنورة الوفاة سنة 690 (89–90 سنة) الكوفة مواطنة الخلافة الراشدة اللقب الأوسي الأنصاري مشكلة صحية عمى الحياة العملية الطب…

此條目没有列出任何参考或来源。 (2018年9月17日)維基百科所有的內容都應該可供查證。请协助補充可靠来源以改善这篇条目。无法查证的內容可能會因為異議提出而被移除。 此條目需要精通或熟悉相关主题的编者参与及协助编辑。 (2018年3月17日)請邀請適合的人士改善本条目。更多的細節與詳情請參见討論頁。 Yunnan Lvshan Landscape車隊資訊UCI代碼YUN注册地中国成立年份2014項目公…

American poet (1928–1974) For the singer, see Ann Sexton. Anne SextonAnne Sexton photographed by Elsa DorfmanBornAnne Gray Harvey[1](1928-11-09)November 9, 1928Newton, Massachusetts, U.S.DiedOctober 4, 1974(1974-10-04) (aged 45)Weston, Massachusetts, U.S.OccupationPoetEducationBoston UniversityLiterary movementConfessional poetrySpouseAlfred Sexton (1948–1973)Children2, including Linda Gray Sexton Anne Sexton (born Anne Gray Harvey; November 9, 1928 – October 4, 1974) was an A…

1919 film by Hugh Ford Secret ServiceLobby artDirected byHugh FordJoe Boyle (assistant director)Written byBeulah Marie Dix (scenario)Based onSecret Service (play)by William GilletteProduced byAdolph ZukorJesse LaskyStarringRobert WarwickWanda HawleyCinematographyWilliam MarshallDistributed byParamount PicturesRelease date June 15, 1919 (1919-06-15) Running time60 minutes; 6 reelsCountryUnited StatesLanguageSilent (English intertitles) Secret Service is a lost[1] 1919 Ameri…

Gibraltar ten centimos Queen Victoria stamp of 1889 (note the use of Spanish currency on a British stamp) This is a survey of the postage stamps and postal history of Gibraltar. Gibraltar is a British overseas territory located at the entrance to the Mediterranean Sea overlooking the Strait of Gibraltar. The territory covers 6.843 square kilometres (2.642 sq mi) and shares a land border with Spain to the north. Gibraltar has historically been an important base for the British Armed For…

Tanzania en los Juegos Olímpicos Bandera de TanzaniaCódigo COI TANCON Comité Olímpico de TanzaniaMedallas 0 2 0 2 Historia olímpicaJuegos de verano 1964* • 1968 • 1972 • 1976 • 1980 • 1984 • 1988 • 1992 • 1996 • 2000 • 2004 • 2008 • 2012 • 2016 • 2020 • 2024 • *Participó como Tanganica[editar datos en Wikidata…

Kementerian Unifikasi통일부統一部Tong-il-buLogoInformasi lembagaDibentuk1 Maret 1969 (1969-03-01)Nomenklatur lembaga sebelumnyaBadan Unifikasi Nasional (1969-1998)Wilayah hukumKorea SelatanKantor pusatJongno-gu, SeoulMenteriCho Myung-gyun, Menteri UnifikasiWakil MenteriChun Hae-sung, Wakil MenteriLembaga indukPemerintah Korea SelatanLembaga bawahanBadan Dialog Antar-KoreaInstitut Pendidikan UnfikasiPusat Dukungan Pemukiman untuk Pengungsi Korea UtaraBadan Konsultasi Pertukaran dan Ker…